Neuroscientists study how neuron network activity is affected in the early stages of Alzheimer’s Disease
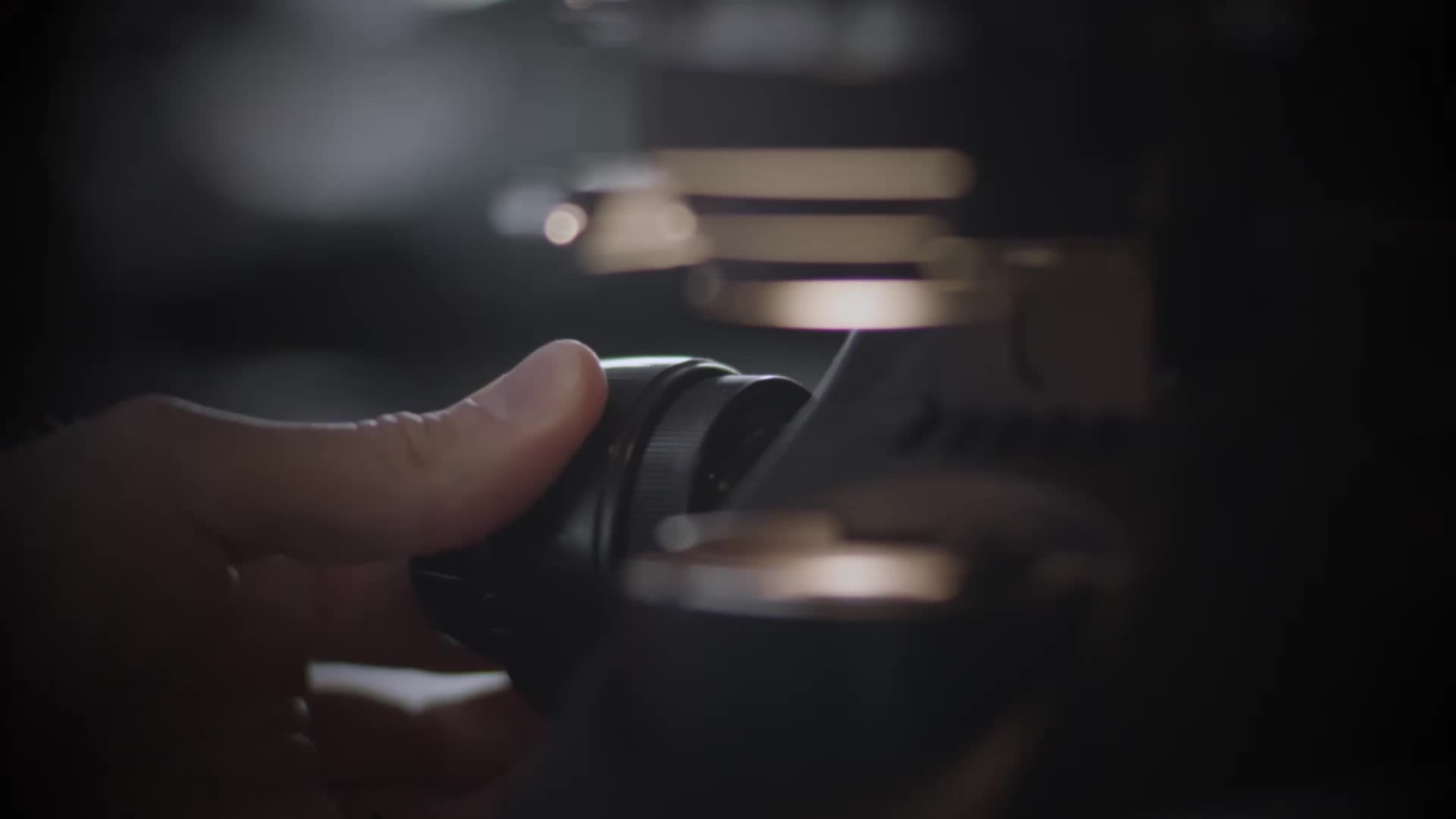
Most people are familiar with the devastating effects of Alzheimer's disease. However, many are not aware that the symptoms and underlying biology of Alzheimer's don't take place suddenly, but instead progress over a long time.
One of the earliest stages of Alzheimer's is the prodromal phase. While the classic behavioral hallmarks of the disease - such as errors in memory, thinking, and reasoning - may not be present during this stage, many people will experience other, more subtle differences, such as sleep disturbances.
Even though obvious Alzheimer's symptoms are absent, the prodromal phase is characterized by classic biological markers of the disease in the brain, such as toxic protein build-up and neuron network hyperactivity.
Because cognition remains largely intact despite these changes in the brain, researchers believe homeostatic mechanisms might keep the brain functioning in the prodromal stage of the disease.
However, what these mechanisms are or how they work are not yet well understood. In the heart of Europe - at the VIB-KU Leuven Center for Brain & Disease Research - several labs have been studying this.
Pictured below: Neuroscientists working at the VIB-KU Center for Brain & Disease Research.
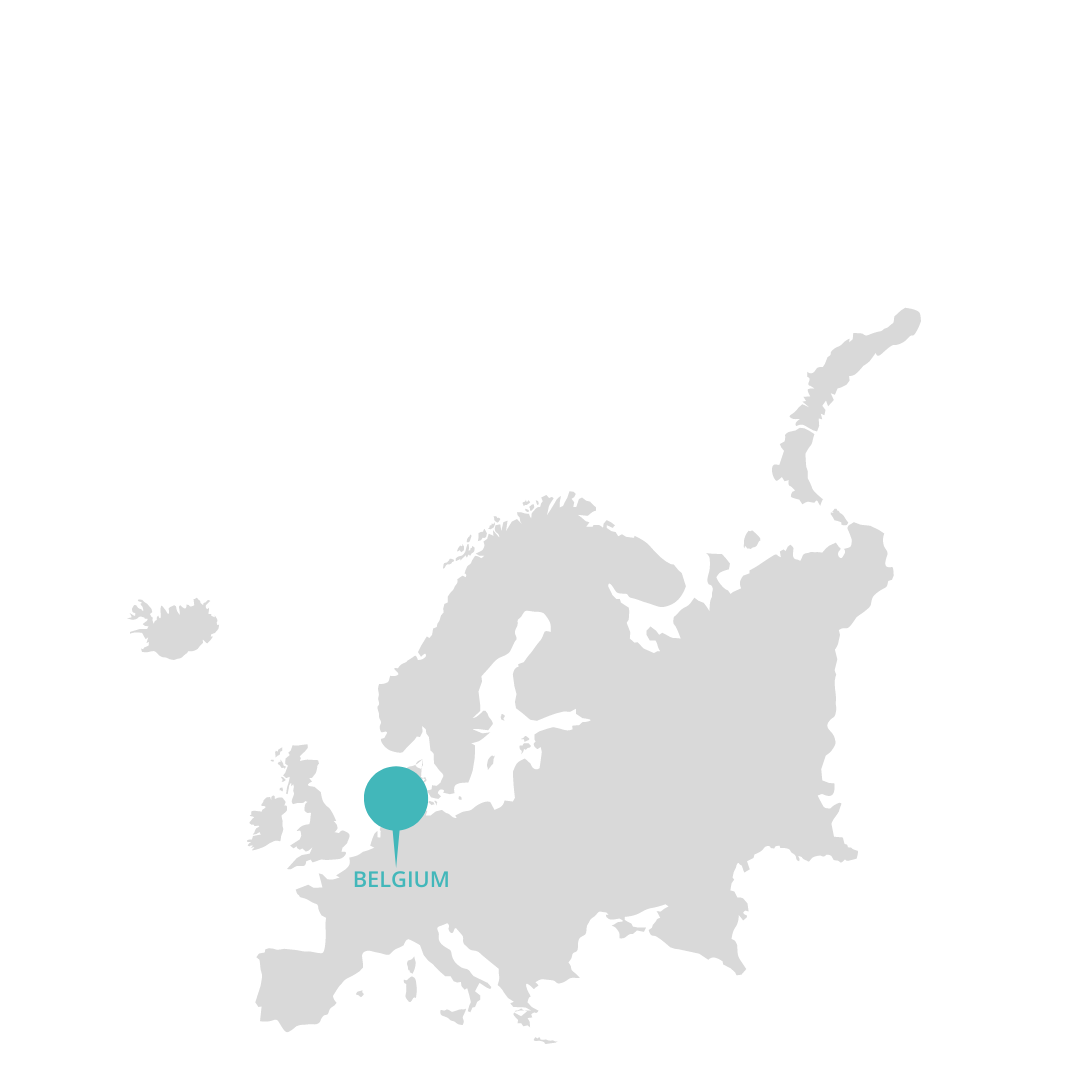
The VIB-KU Leuven Center for Brain & Disease Research in Leuven, Belgium.
The VIB-KU Leuven Center for Brain & Disease Research in Leuven, Belgium.
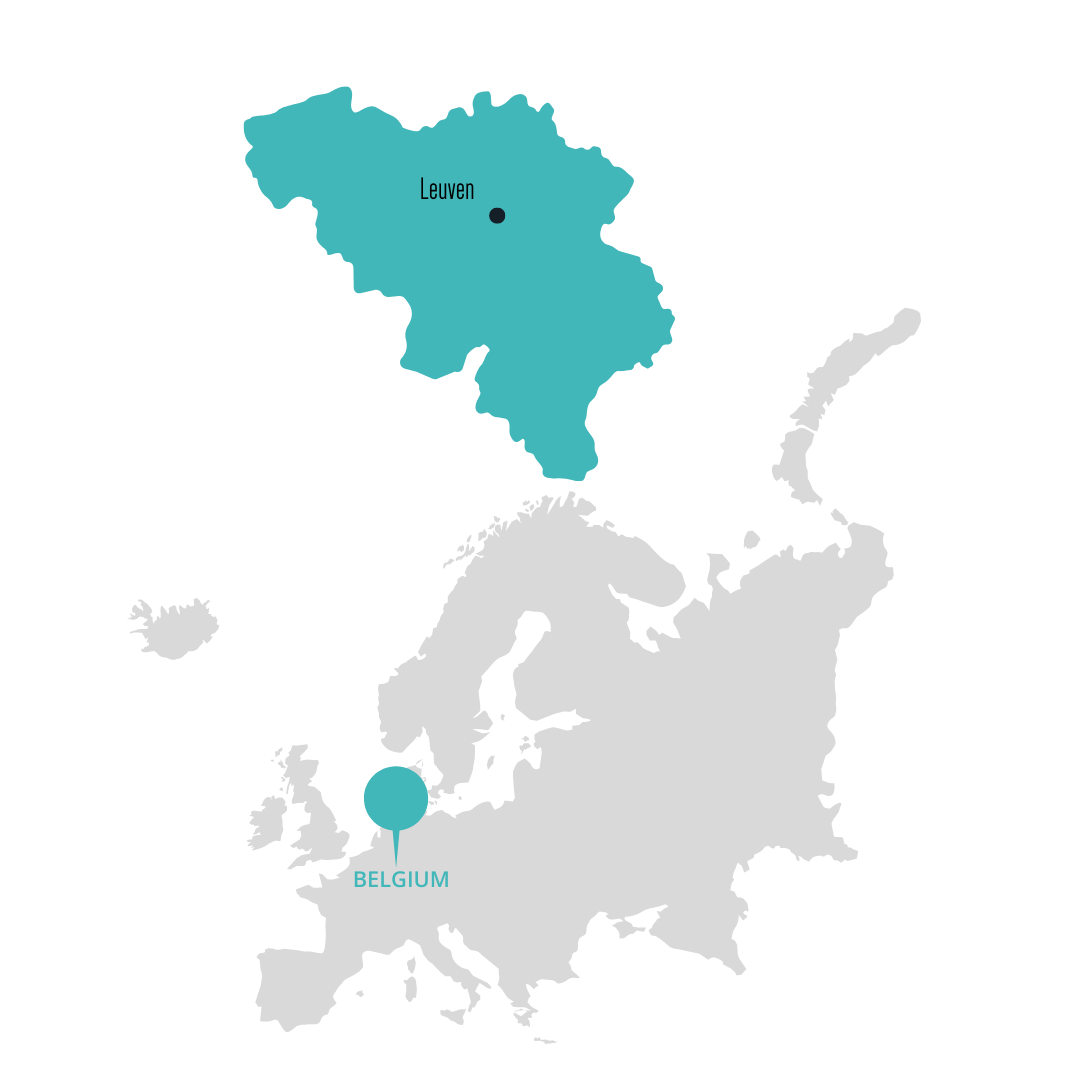
The VIB-KU Leuven Center for Brain & Disease Research in Leuven, Belgium.
The VIB-KU Leuven Center for Brain & Disease Research in Leuven, Belgium.
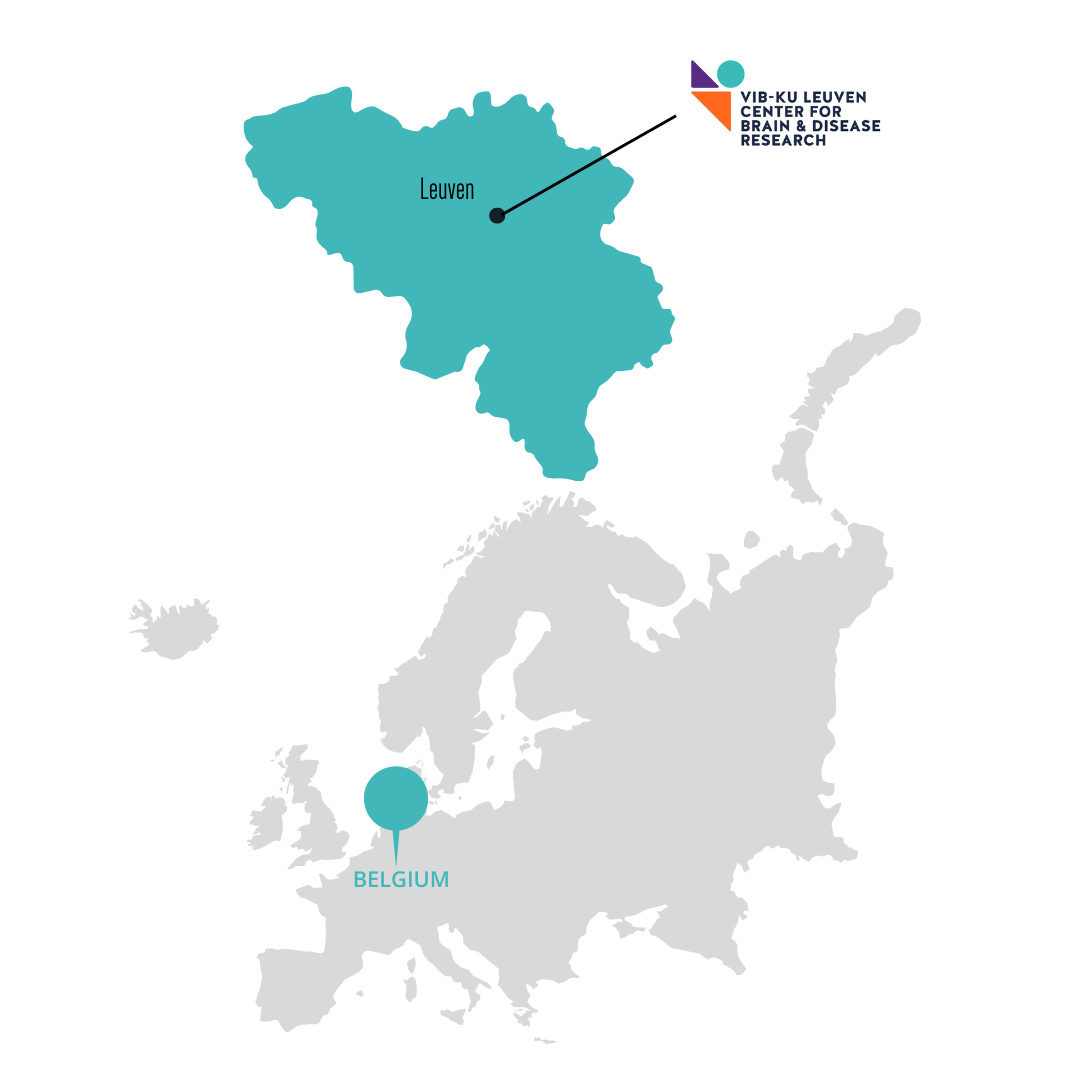
The VIB-KU Leuven Center for Brain & Disease Research in Leuven, Belgium.
The VIB-KU Leuven Center for Brain & Disease Research in Leuven, Belgium.
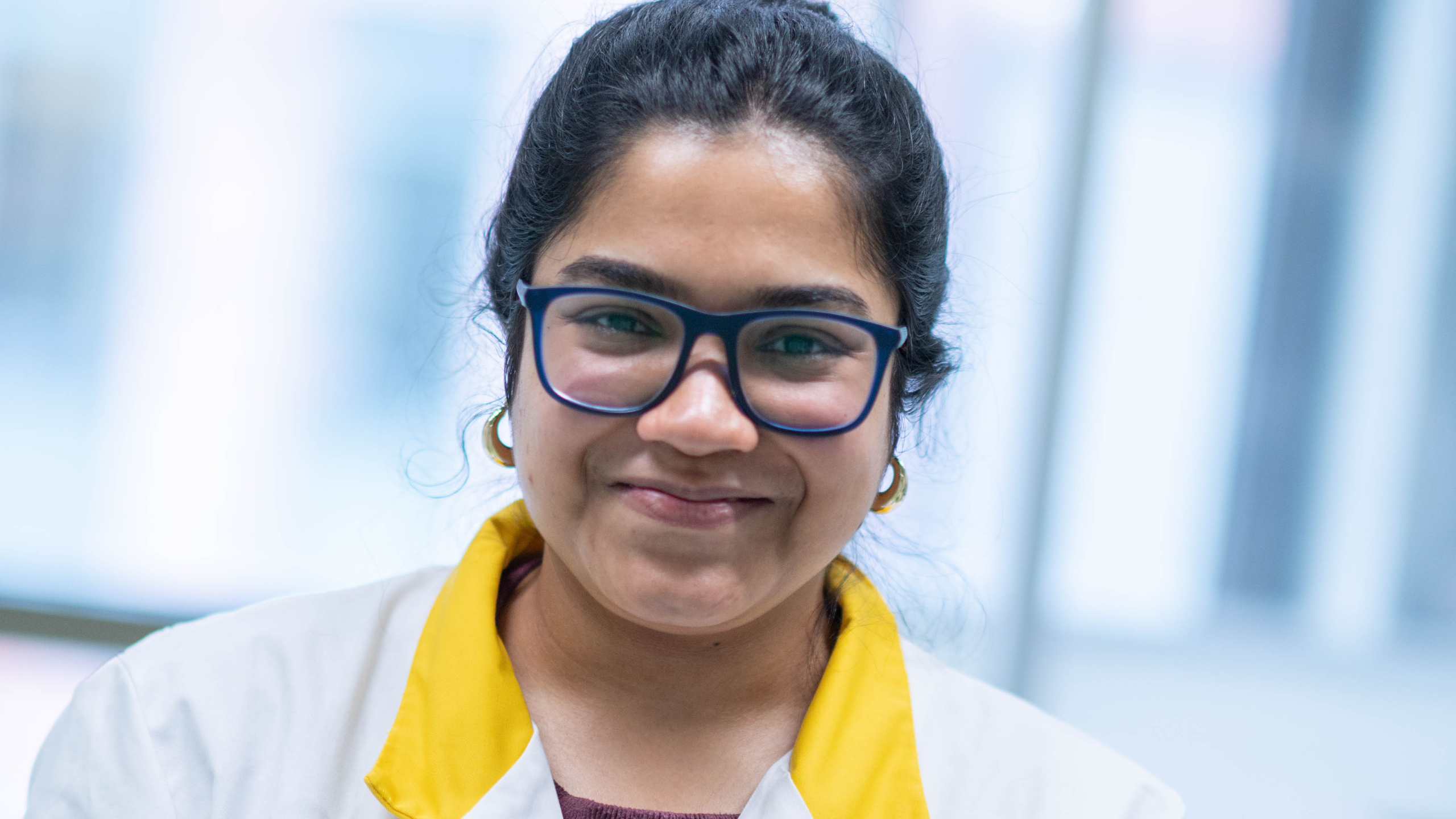
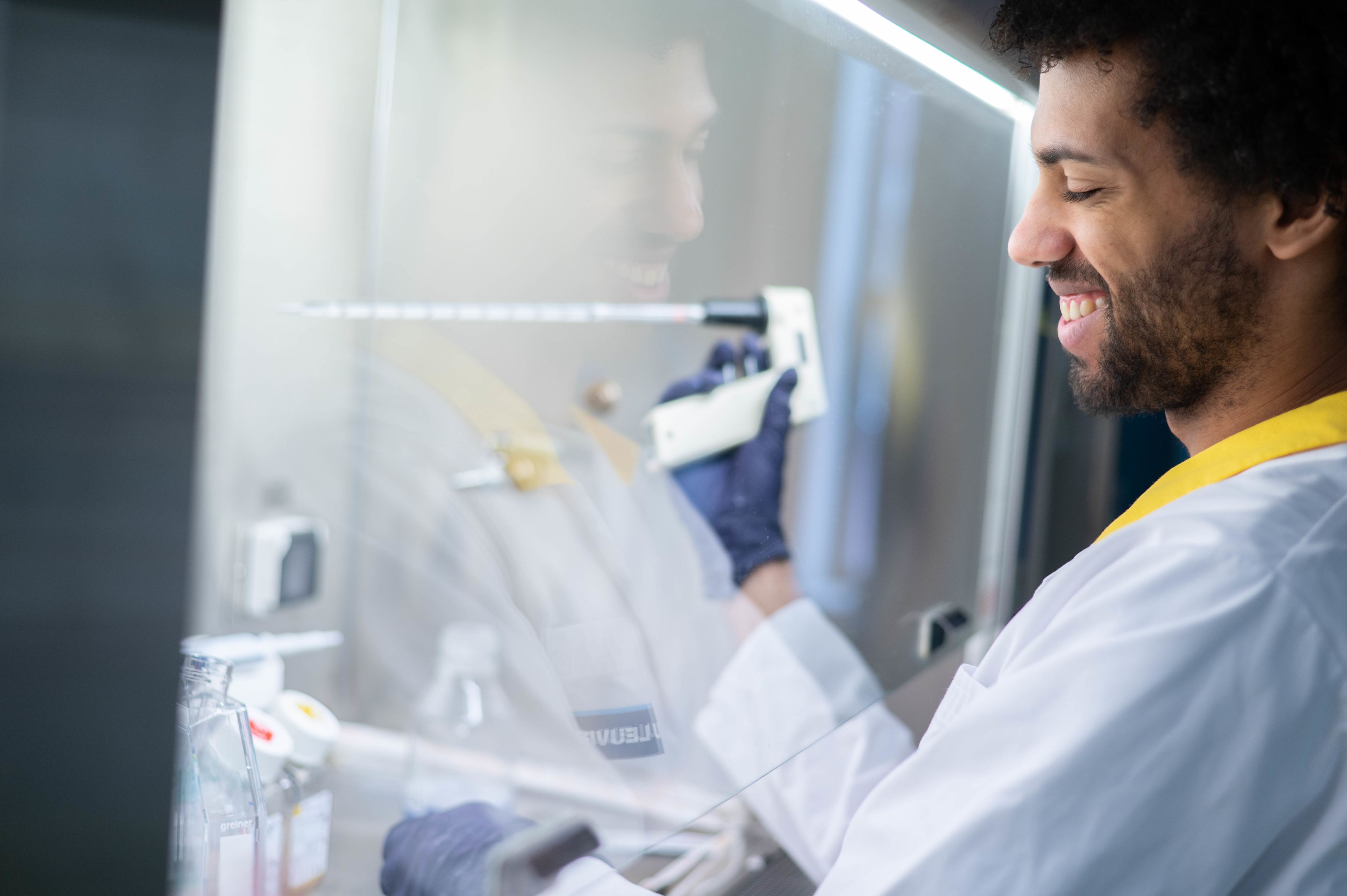
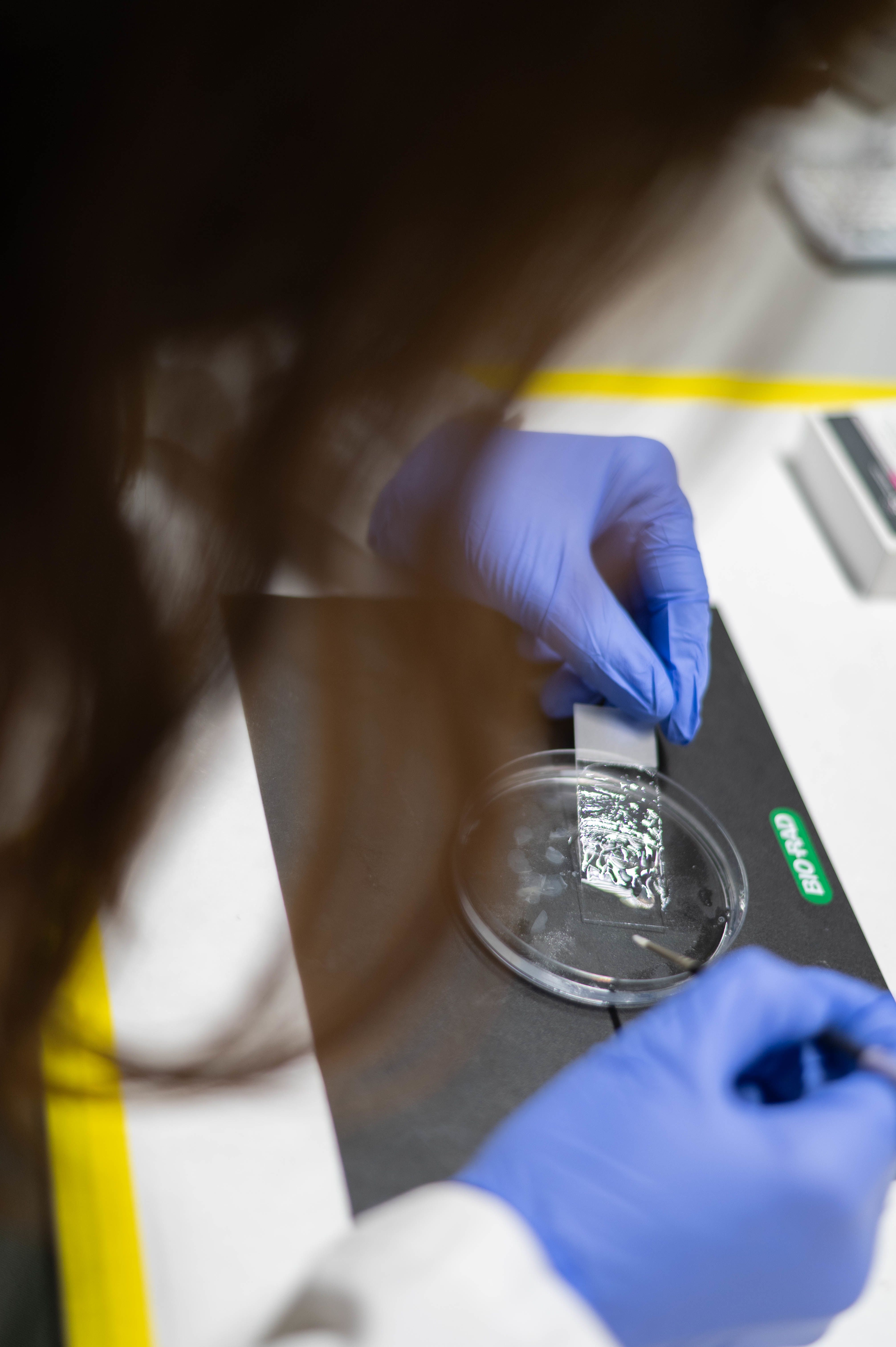
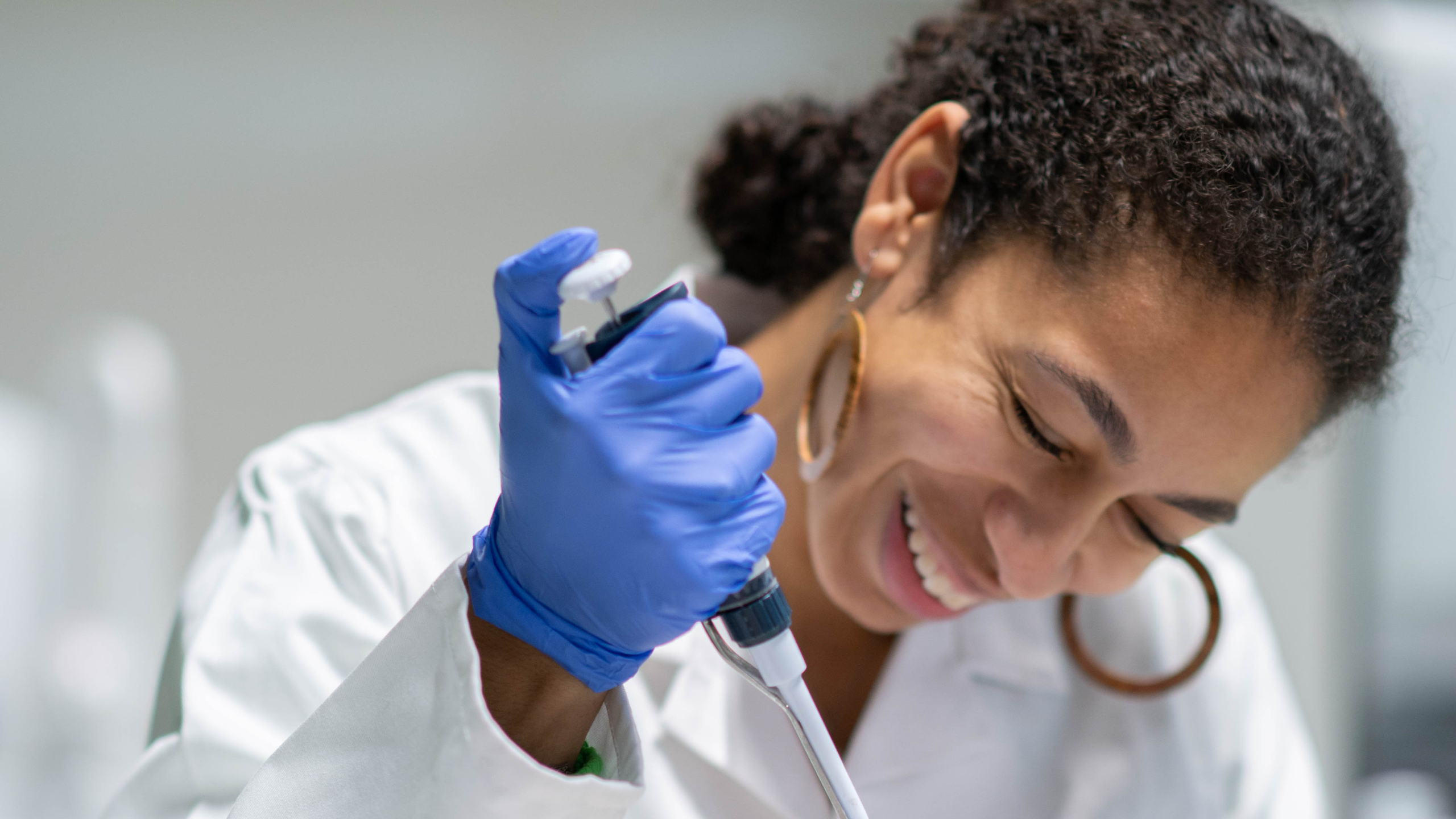
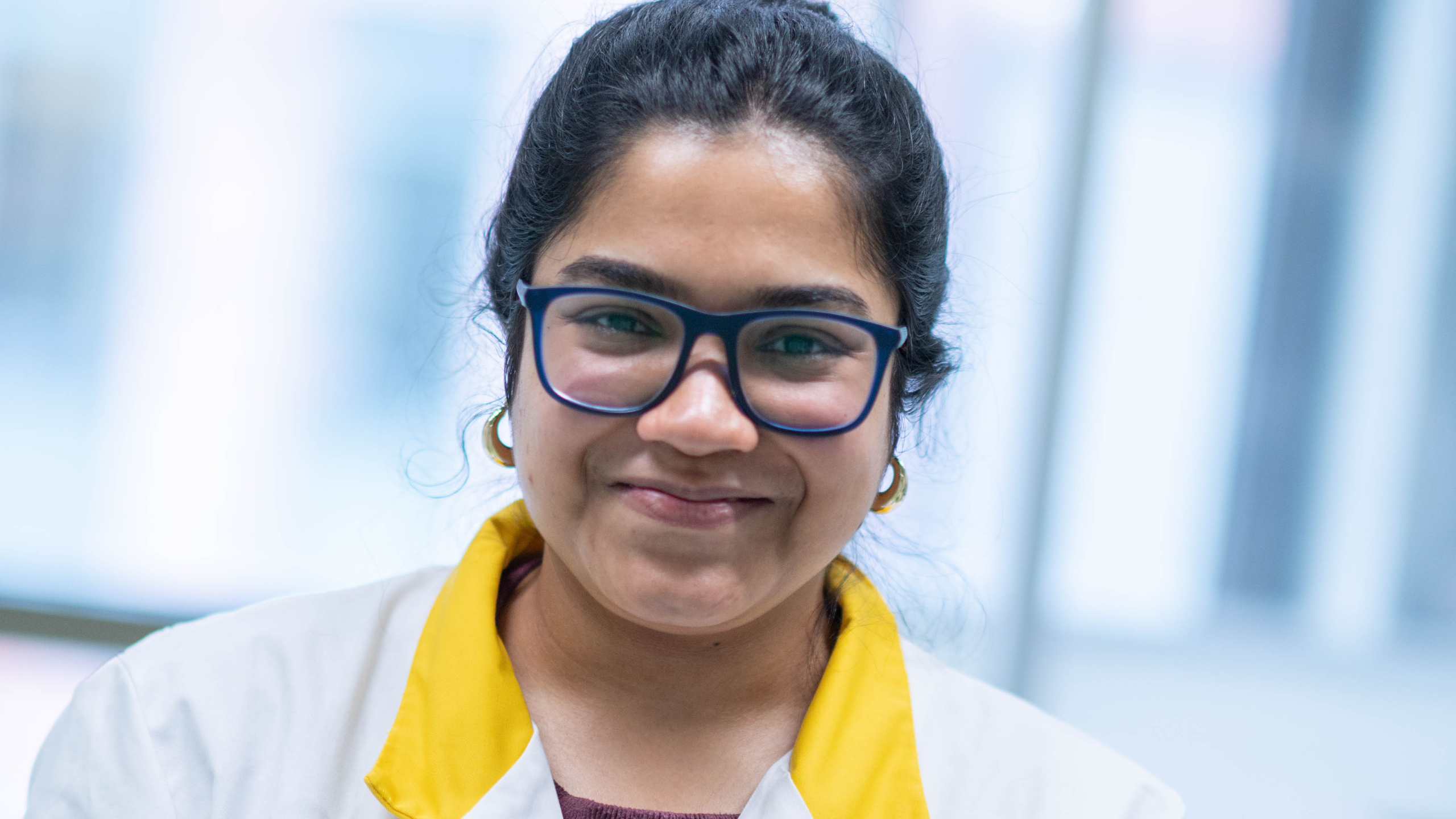
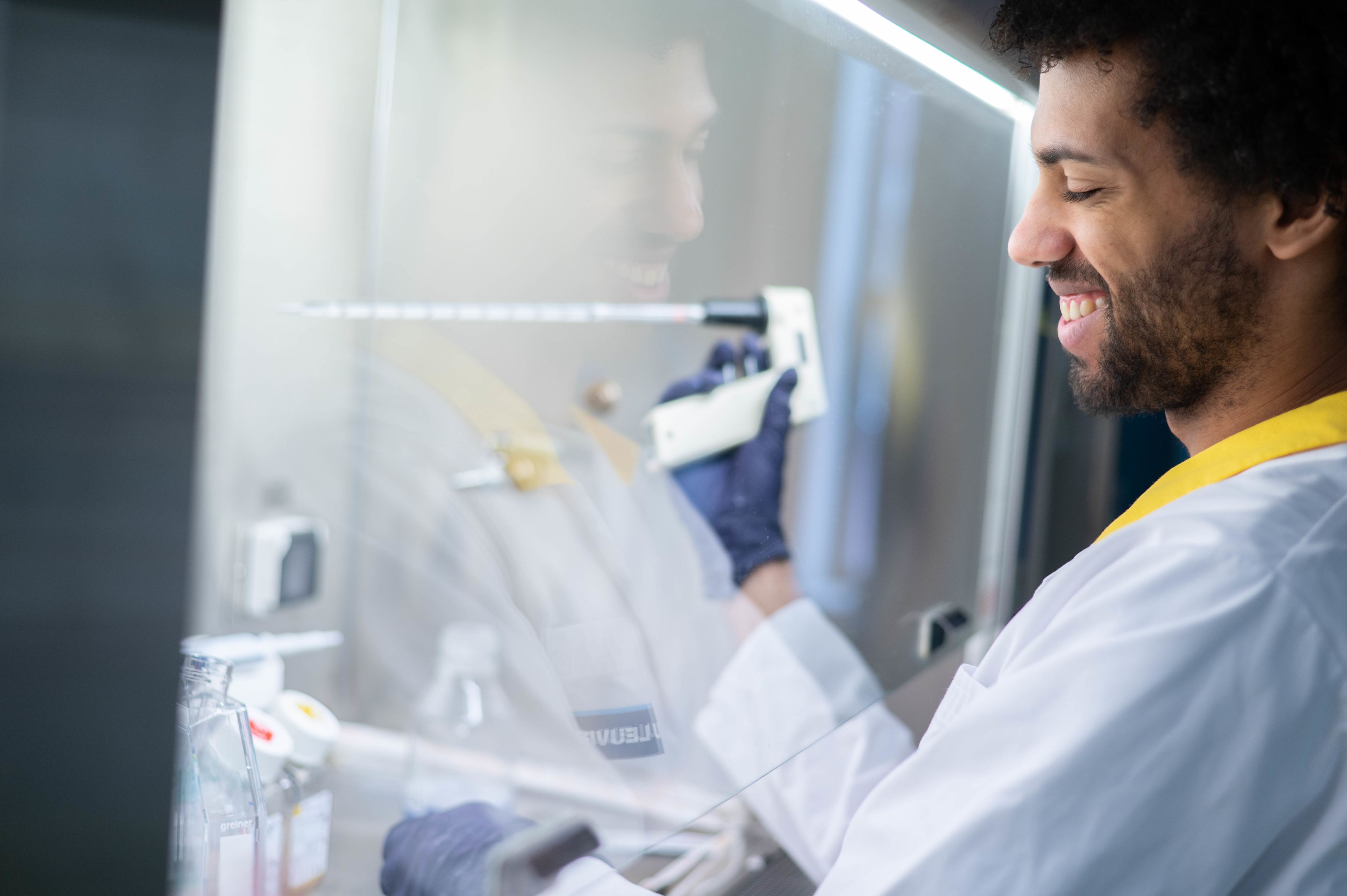
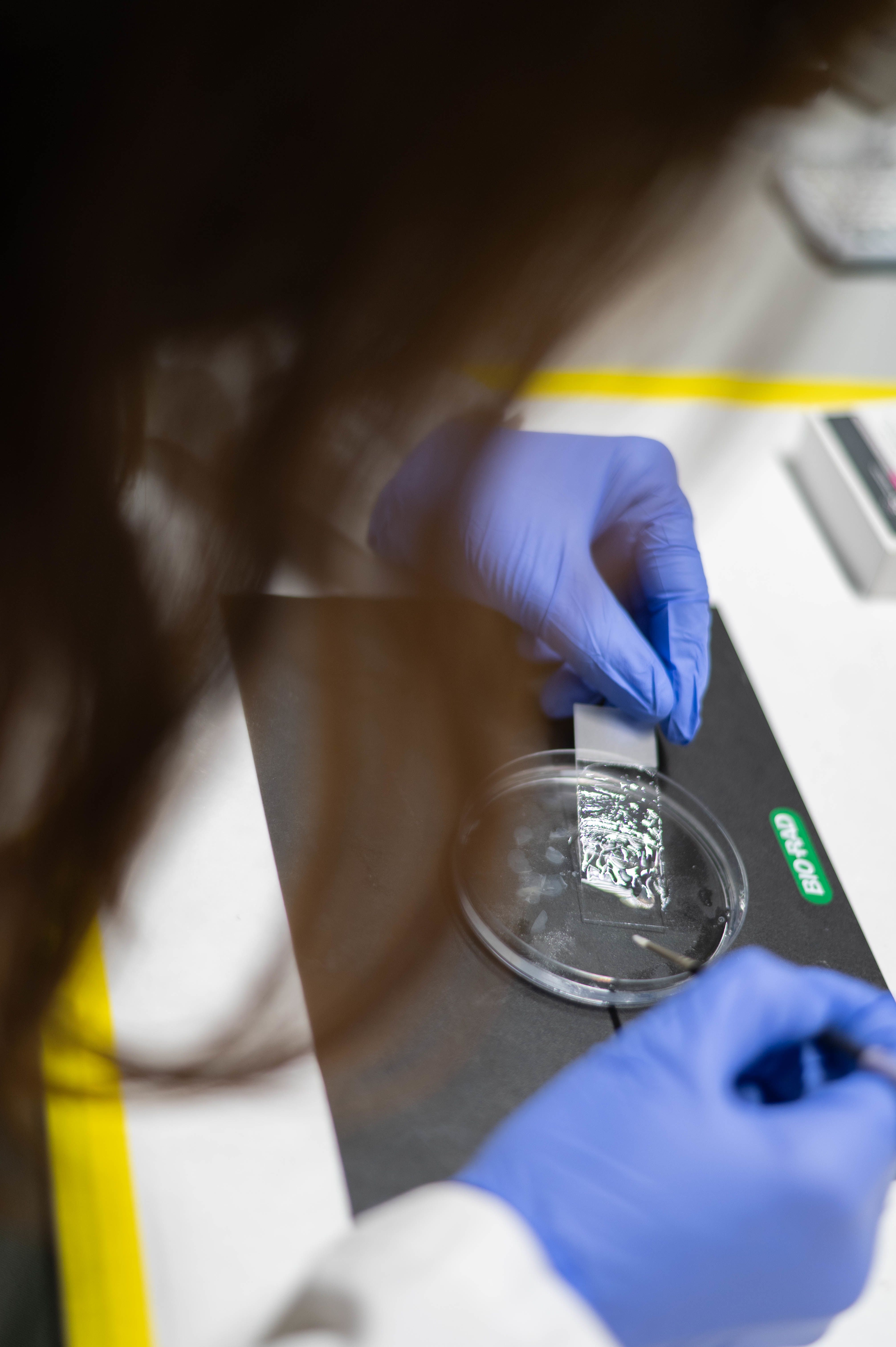
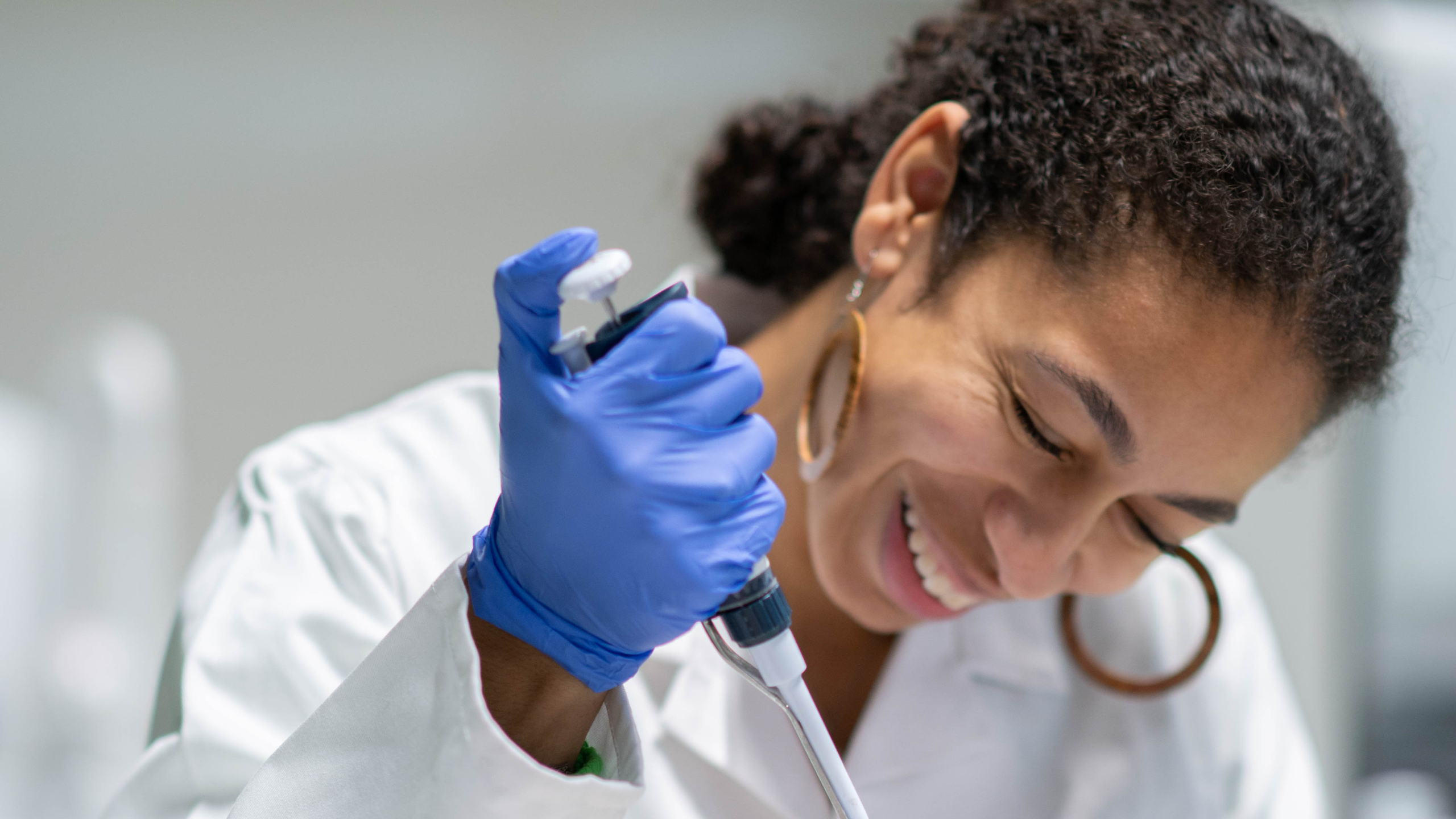
Meet Sara Calafate, a former postdoctoral researcher at the VIB-KU Leuven Center for Brain & Disease Research who aims to learn more about the brain's protective mechanisms during the early stages of Alzheimer's disease.
I sit down with Sara following the publication of her latest publication - in Nature Neuroscience, no less - to find out more about the research she's been working on.
"I started my postdoc in the labs of Joris De Wit and Bart De Strooper in 2016," Sara shares with me over video call, from the comfort of her home in Portugal, having since moved on to do a second postdoc at the ICVS Life and Health Sciences Research Institute. "From the start, I was really interested in synapse function and dysfunction in the context of Alzheimer's disease. But how I stumbled onto this specific research project was a complete surprise."
Sara's postdoc journey started by recording the electrical activity in neurons from the CA1 area of the hippocampus in Alzheimer's mouse models at different ages.
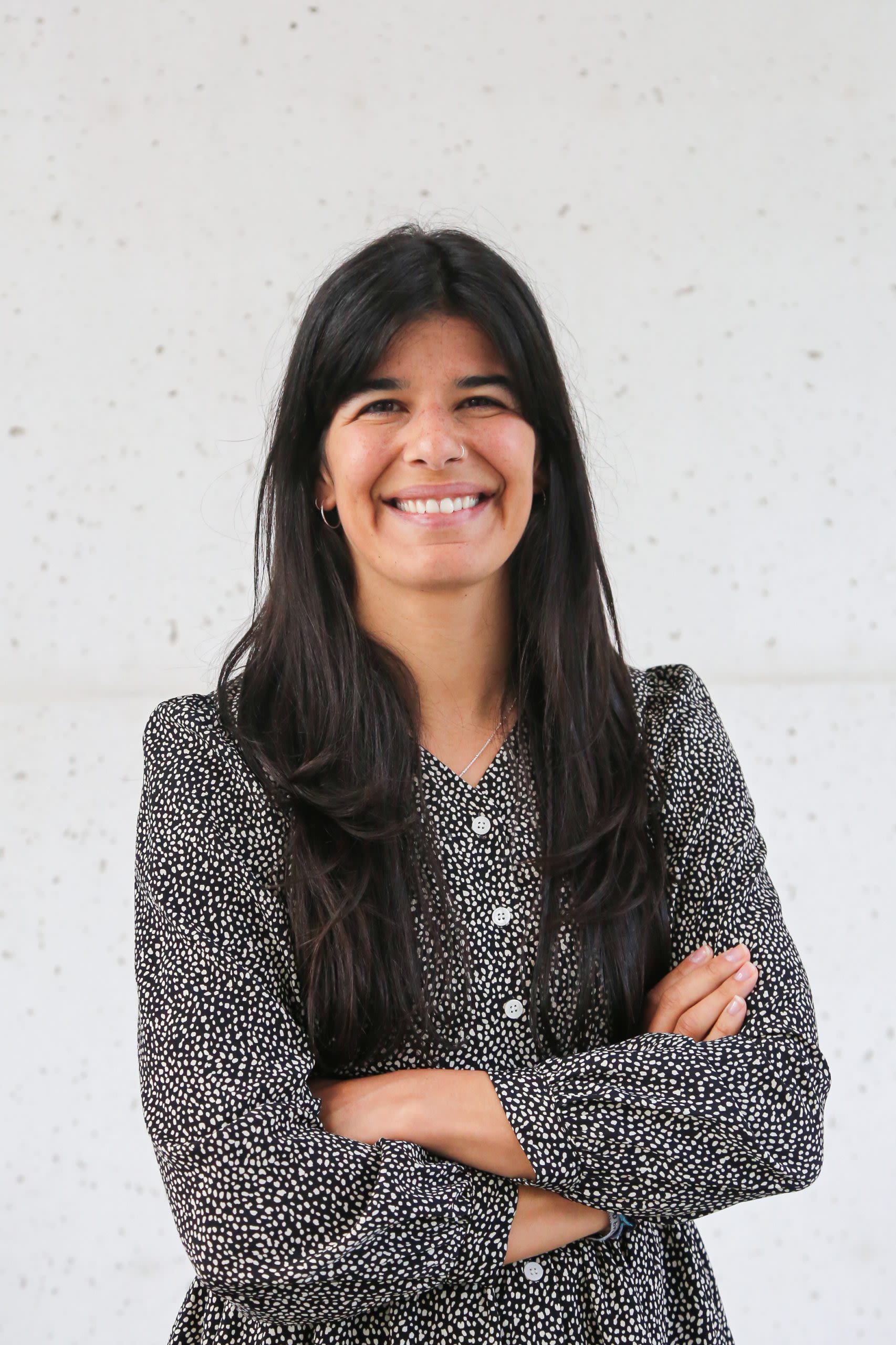
Sara Calafate, former postdoctoral researcher at the VIB-KU Leuven Center for Brain & Disease Research.
Sara Calafate, former postdoctoral researcher at the VIB-KU Leuven Center for Brain & Disease Research.
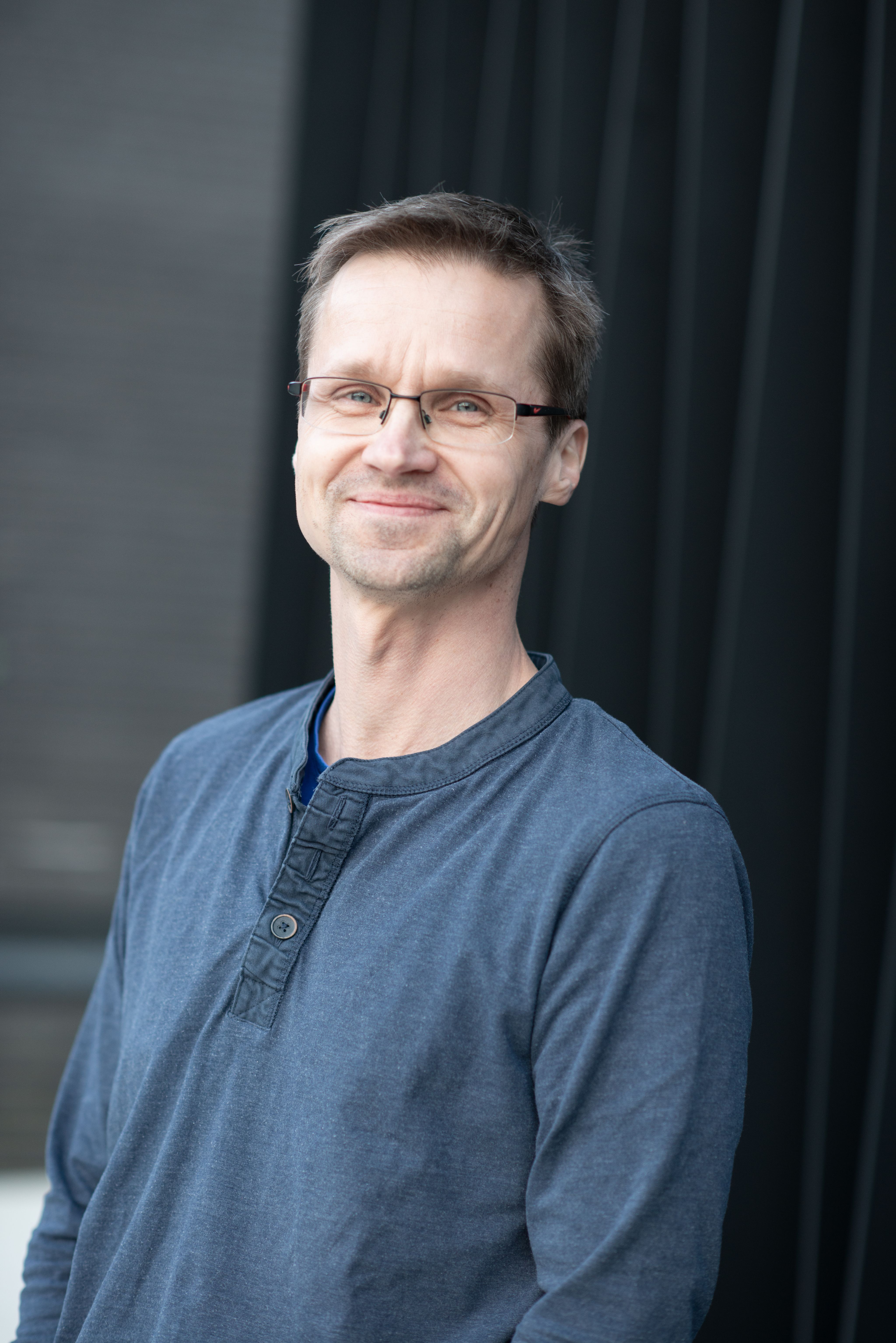
Keimpe Wierda, head of electrophysiology unit at the VIB-KU Leuven Center for Brain & Disease Research.
Keimpe Wierda, head of electrophysiology unit at the VIB-KU Leuven Center for Brain & Disease Research.
To measure electrical activity in neurons, Sara sought help from Keimpe Wierda, expert in electrophysiology at the Center.
"At the beginning of my study, he was also a postdoctoral researcher in Joris' lab, just like me," Sara recalls. "These days, he now runs his own electrophysiology expertise unit!"
Keimpe supports the work of numerous labs at the Center with his electrophysiology know-how. You can take a tour of his set-up below!
But what exactly is electrophysiology technology, and how does it work? "The round structures are dozens of neurons in a section of brain tissue," Keimpe explains, indicating the picture on the bottom-left. "To measure the excitability of one individual neuron, we approach that specific cell with a glass capillary and establish a direct contact with the internal side of the cell. In this way, we can excite the selected neuron with electrical stimulation, while the surrounding cells remain unaffected."
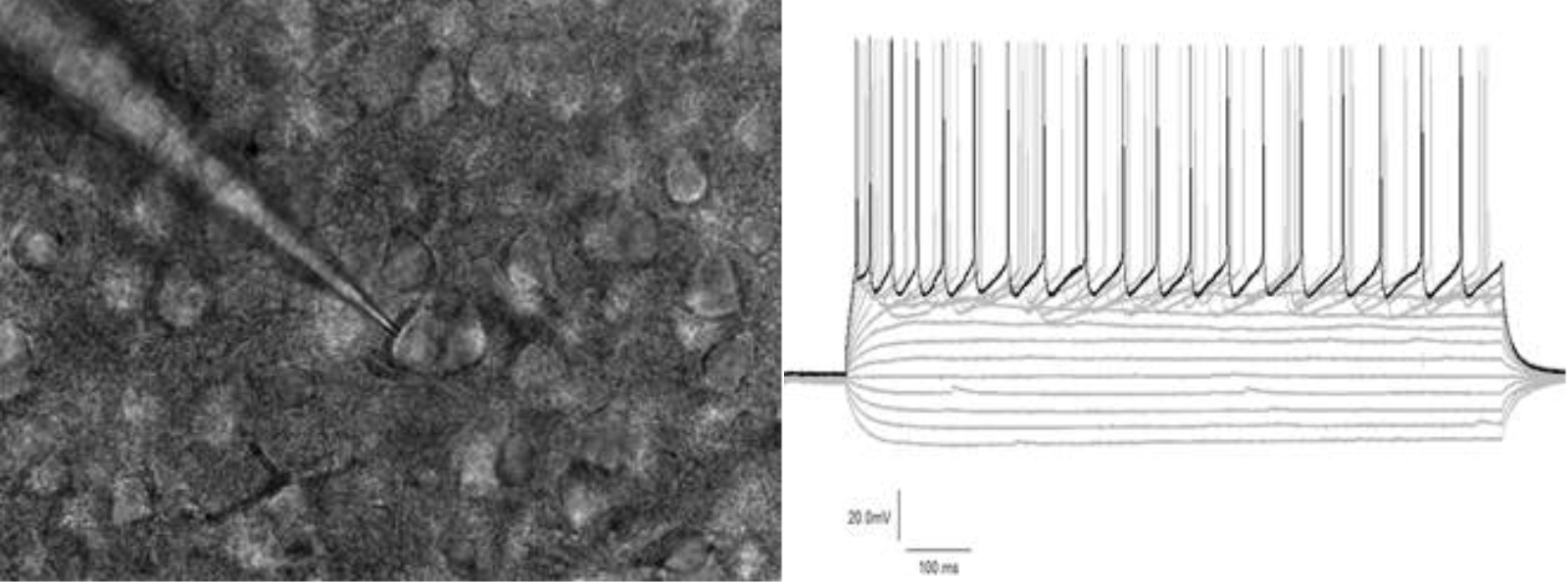
Left: Section of brain tissue. Right: Electrophysiology measurements.
Left: Section of brain tissue. Right: Electrophysiology measurements.
"When exciting the neuron with enough electrical stimulation, it will start to generate these upward peaks, which we call action potentials," Keimpe continues, pointing out the spikes in the above-right diagram. "These action potentials are the main communication method for each neuron in a neuronal network to interact with other connected neurons."
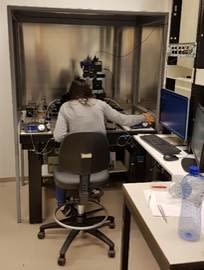
This is a picture of Sara in 2016-2017 at the start of her postdoc in the labs of Joris de Wit and Bart De Strooper. In this picture, Sara is starting to learn the techniques required to do electrophysiological experiments by herself.
This is a picture of Sara in 2016-2017 at the start of her postdoc in the labs of Joris de Wit and Bart De Strooper. In this picture, Sara is starting to learn the techniques required to do electrophysiological experiments by herself.
Keimpe goes on to talk about his involvement in Sara's project. "Sara was one of the first scientists that learned to do electrophysiological experiments under my guidance," Keimpe recalls. "It was clear from the start that her project would require a lot of electrophysiology and she was very keen and enthusiastic to dive into this somewhat niche world in neuroscience. Sara did a tremendous job in finding her way in this new world and her heroic efforts made me proud."
While recording the electrical activity in neurons from the CA1 region in Alzheimer's mouse models across varying ages, Sara noticed unusual electrical activity at specific time points.
Sara decided to compare the unexpected electrical activity with molecular profiling data that was available. "Unexpectedly, a gene called Pmch showed up in these analyses. It was a surprise because this gene was not usually linked to synapse function." Sara continues. Pmch encodes melanin-concentrating hormone (MCH), which is made in neurons in the lateral hypothalamic area (LHA). These neurons connect to the CA1 region of the hippocampus, which is a key part of the brain involved in sleep and memory processes.
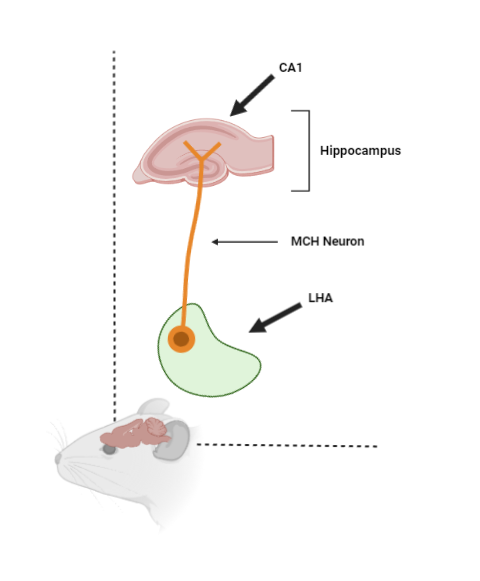
A cartoon showing how MCH neurons reside in the LHA area and project to the CA1 region of the hippocampus.
A cartoon showing how MCH neurons reside in the LHA area and project to the CA1 region of the hippocampus.
"We didn't pay much attention to it at first," Sara admits. "But then I remember getting an excited email from Joris late one evening, who'd been digging into it and suggested that we start to focus on it more." I ask Joris de Wit - Sara's postdoctoral supervisor and study co-lead - how he came to realize this might be an interesting avenue to pursue.
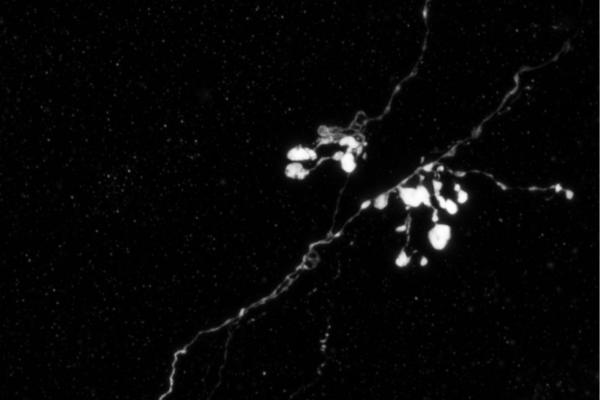
An MCH axon (a fiber that connects neurons so that they can communicate) residing in the brain of a mouse model of Alzheimer's disease. Image courtesy of Sara Calafate.
An MCH axon (a fiber that connects neurons so that they can communicate) residing in the brain of a mouse model of Alzheimer's disease. Image courtesy of Sara Calafate.
"MCH-expressing neurons were already known to be active during sleep and involved in hippocampus-dependent memory," Joris highlights. "But after noticing the parallels between existing molecular data and Sara's electrophysiology recordings, we realized that by studying MCH neurons, we might be able to understand more about the homeostatic mechanisms involved in the prodromal phase of Alzheimer's."
Sara and colleagues went on to do several experiments to understand more about the role of melanin-concentrating hormone (MCH) in early Alzheimer's.
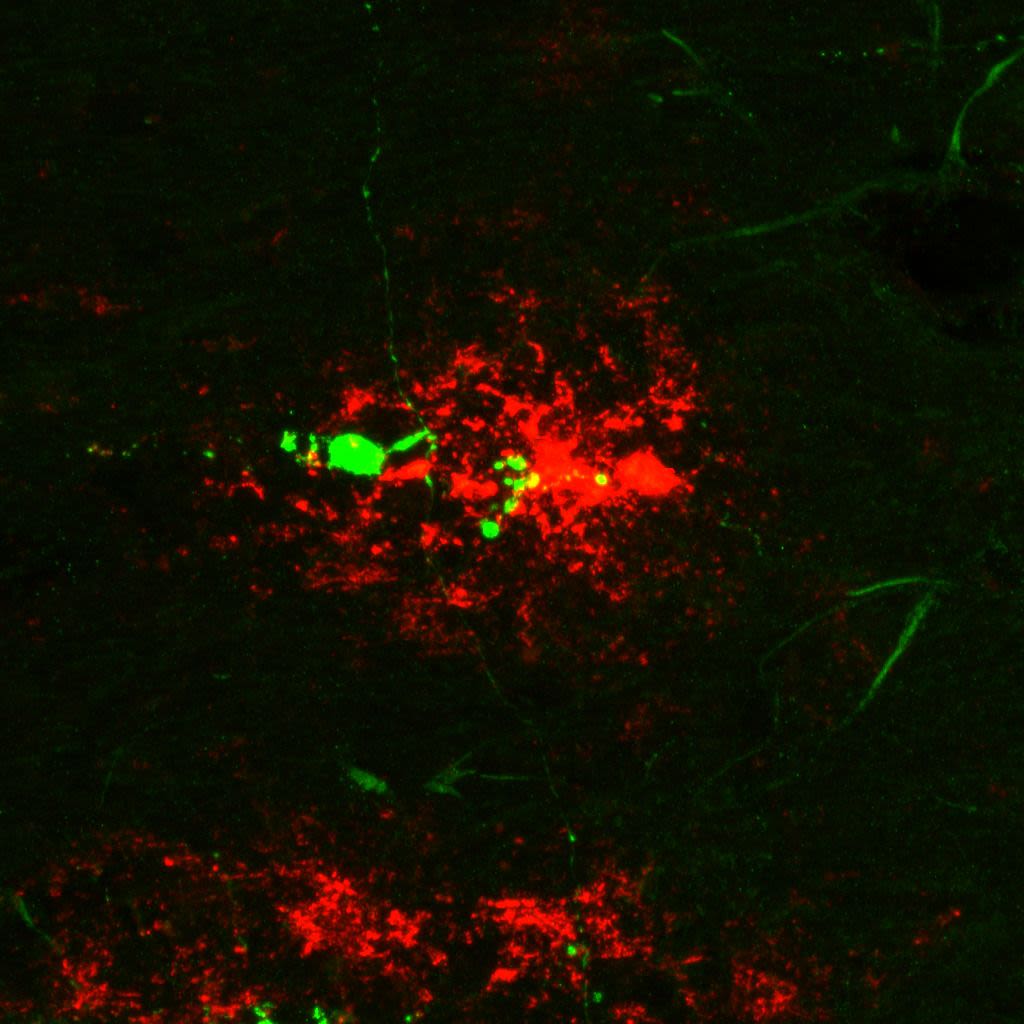
α-beta plaque shown in red, and an MCH axon shown in green. Image courtesy of Sara Calafate.
α-beta plaque shown in red, and an MCH axon shown in green. Image courtesy of Sara Calafate.
The researchers found that in both an Alzheimer's mouse model and in human brain tissue of Alzheimer patients, the connections of MCH neurons are damaged.
Promisingly, these scientists also found that by introducing an MCH peptide (a short string of amino acids, the building blocks of proteins) into hippocampal brain slices from the Alzheimer’s disease mouse model, they could reverse the detrimental hyperactivity in the hippocampal neuron network.
I was curious to know what these findings might mean for the future of Alzheimer's disease research.
I ask Bart De Strooper – another study co-lead Scientific Director of the UK Dementia Research Institute, who has been studying Alzheimer's at the VIB-KU Leuven Center for Brain & Disease Research for over 30 years - to share his thoughts.
Sara Calafate shares a bite-sized summary of her latest paper: "Early alterations in the MCH system link aberrant neuronal activity and sleep disturbances in a mouse model of Alzheimer’s disease | Nature Neuroscience" (Calafate et al., 2023, Nature Neuroscience).
"All in all, our findings show the MCH system to be vulnerable in the early stages of Alzheimer's disease," Bart explains. "In addition, the lateral hypothalamic area has not been previously demonstrated to be involved in the underlying neurobiology of Alzheimer's disease. This provides exciting avenues for research into the disease moving forward."
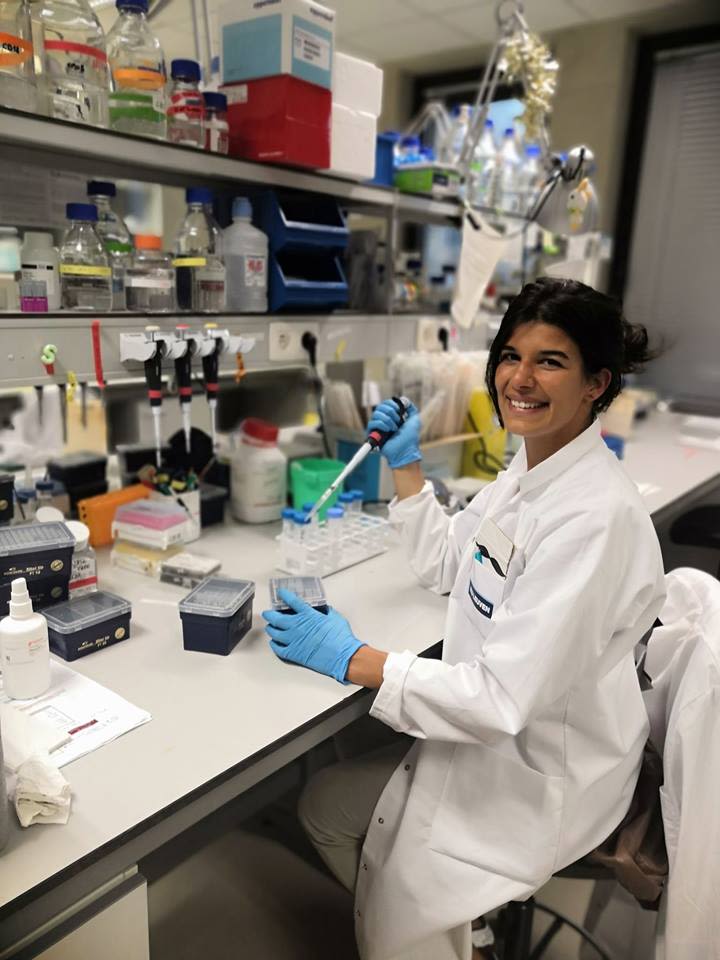
Sara Calafate working in the lab as a postdoctoral researcher at the VIB-KU Leuven Center for Brain & Disease Research.
Sara Calafate working in the lab as a postdoctoral researcher at the VIB-KU Leuven Center for Brain & Disease Research.
This research project was possible thanks to fantastic collaborations with labs across Belgium, Portugal, Israel, Switzerland, and the UK. Furthermore, the team is grateful for their cooperation with Dietmar Thal, a neuropathologist at the neighboring UZ Leuven hospital who provided patient brain samples.